How Does Heat Energy Continue to Be Produced Inside the Earth to Keep It Relatively Hot
3.3 Earth's Interior Heat
Earth Gets Hotter the Deeper You Go
Earth's temperature increases with depth, but not at a uniform rate (Figure 3.11). Earth's geothermal gradient is 15° to 30°C/km within the crust. It then drops off dramatically through the mantle, increases more quickly at the base of the mantle, and then increases slowly through the core. The temperature is approximately 1000°C at the base of the crust, around 3500°C at the base of the mantle, and approximately 6,000°C at Earth's centre.
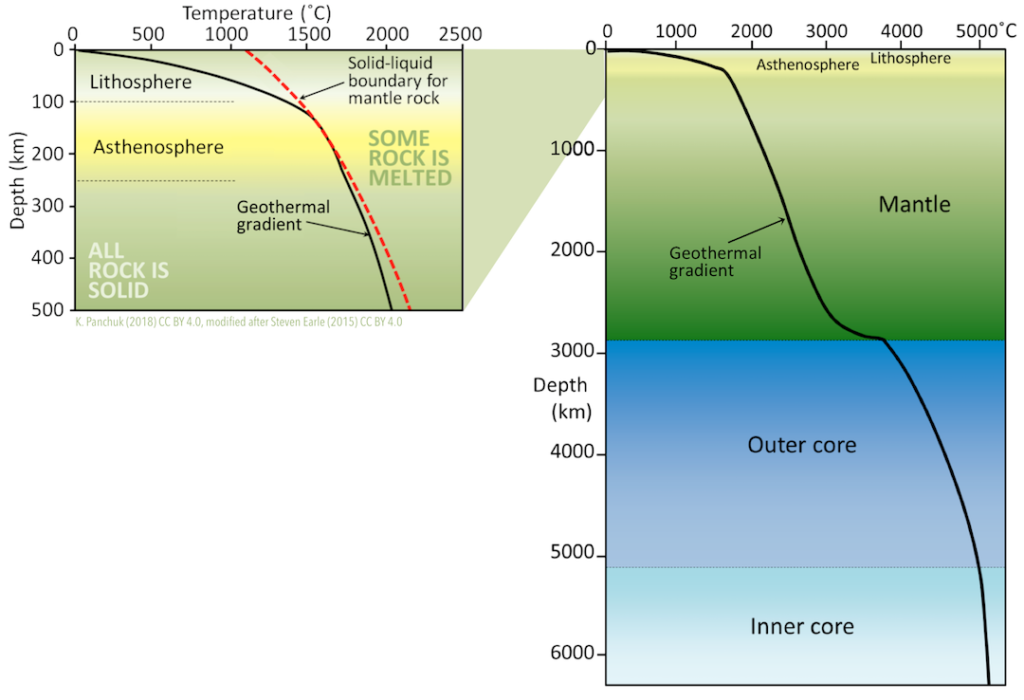
The temperature gradient within the lithosphere varies depending on the tectonic setting. Gradients are lowest in the central parts of continents, higher where plates collide, and higher still at boundaries where plates are moving away from each other.
In spite of high temperatures within Earth, mantle rocks are almost entirely solid. High pressures keep them from melting. The red dashed line in Figure 3.11 (right) shows the minimum temperature at which dry mantle rocks will melt. Rocks at temperatures to the left of the line will remain solid. In rocks at temperatures to the right of the line, some minerals will begin to melt. Notice that the red dashed line goes further to the right for greater depths, and therefore greater pressures. Now compare the geothermal gradient with the red dashed line. The geothermal gradient is to the left of the red line, except in the asthenosphere, where small amounts of melt are present.
Convection Helps to Move Heat Within Earth
The fact that the temperature gradient is much lower in the main part of the mantle than in the lithosphere has been interpreted as evidence of convection in the mantle. When the mantle convects, heat is transferred through the mantle by physically moving hot rocks. Mantle convection is the result of heat transfer from the core to the base of the lower mantle. As with a pot of soup on a hot stove (Figure 3.12), the material near the heat source (the soup at the bottom of the pot) becomes hot and expands, making it less dense than the material above. Buoyancy causes it to rise, and cooler material flows in from the sides. Of course, convection in the soup pot is much faster than convection in the mantle. Mantle convection occurs at rates of centimetres per year.
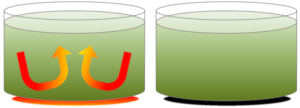
Convection carries heat to the surface of the mantle much faster than heating by conduction. Conduction is heat transfer by collisions between molecules, and is how heat is transferred from the stove to the soup pot. A convecting mantle is an essential feature of plate tectonics, because the higher rate of heat transfer is necessary to keep the asthenosphere weak. Earth's mantle will stop convecting once the core has cooled to the point where there is not enough heat transfer to overcome the strength of the rock. This has already happened on smaller planets like Mercury and Mars, as well as on Earth's moon. When mantle convection stops, the end of plate tectonics will follow.
Models of Mantle Convection
In the soup pot example, convection moves hot soup from the bottom of the pot to the top. Some geologists think that Earth's convection works the same way— hot rock from the base of the mantle moves all the way to the top of the mantle before cooling and sinking back down again. This view is referred to as whole-mantle convection (Figure 3.8, left). Other geologists think that the upper and lower mantle are too different to convect as one. They point to slabs of lithosphere that are sinking back into the mantle, some of which seem to perch on the boundary between the upper and lower mantle, rather than sinking straight through. They also note chemical differences in magma originating in different parts of the mantle— differences that are not consistent with the entire mantle being well stirred. They argue that double-layered convection is a better fit with the observations (Figure 3.13, right). Still others argue that there may be some locations where convection goes from the bottom of the mantle to the top, and some where it doesn't (Figure 3.13, middle).
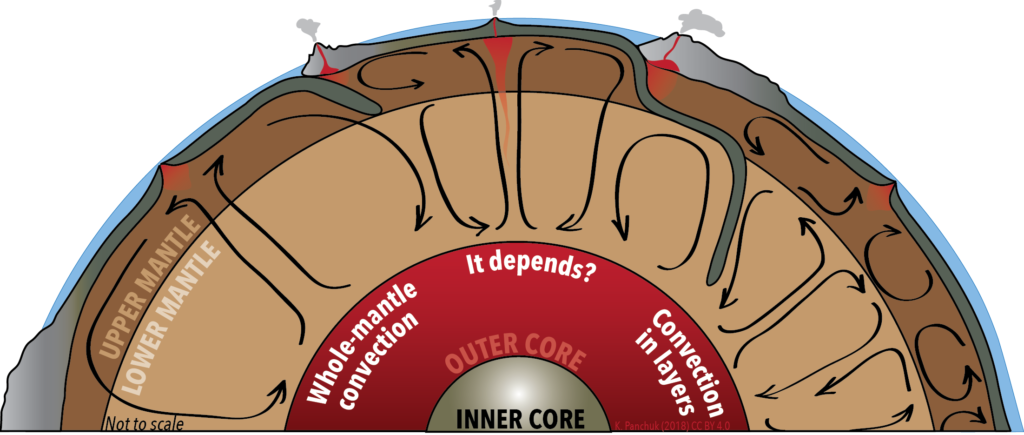
Why Is Earth Hot Inside?
The heat of Earth's interior comes from a variety of sources. These include the heat contained in the objects that accreted to form Earth, and the heat produced when they collided. As Earth grew larger, the increased pressure on Earth's interior caused it to compress and heat up. Heat also came from friction when melted material was redistributed within Earth, forming the core and mantle.
A major source of Earth's heat is radioactivity, the energy released when the unstable atoms decay. The radioactive isotopes uranium-235 (235U), uranium-238 (238U), potassium-40 (40K), and thorium-232 (232Th) in Earth's mantle are the primary source. Radioactive decay produced more heat early in Earth's history than it does today, because fewer atoms of those isotopes are left today (Figure 3.14). Heat contributed by radioactivity is now roughly a quarter what it was when Earth formed.

References
Arevalo, R., McDonough, W., & Luong, M. (2009). The K/U ratio of Earth: Insights into mantle composition, structure and thermal evolution. Earth and Planetary Science Letters, 278(3-4), 361-369. https://doi.org/10.1016/j.epsl.2008.12.023
mewton-woodbeforavy.blogspot.com
Source: https://openpress.usask.ca/physicalgeology/chapter/3-3-earths-interior-heat/
0 Response to "How Does Heat Energy Continue to Be Produced Inside the Earth to Keep It Relatively Hot"
Post a Comment